Nerves: Structure, anatomy and response to injury
1 Orthopaedic Surgery, Queen Elizabeth University Hospital, Glasgow, United Kingdom
Abstract
This chapter reviews the structure of peripheral nerves relevant to clinicians managing peripheral nerve injuries and compression syndromes. Anatomy of the main nerves innervating the hand is described. The response of nerve to injury including conduction block and axonal degeneration is explained, together with the potential for axonal regeneration.
Introduction
The hand has multiple functions including being an organ of information, an organ of accomplishment, and an organ of expression [1]. Central to all these functions are the nerves to the central nervous system which convey sensory information to the central nervous system and control the movement. Damage to nerves by injury or compression therefore has a profound effect on function of the hand. Nerves only have a limited capacity to recover after injury. It is therefore important to understand the structure of nerves and their response to injury in order to provide optimal management to maximize recovery whenever possible.
Structure of peripheral nerves
Peripheral nerves function to pass sensory information from the periphery to the central nervous system (CNS) and information to control movement from the CNS to muscles. This information is conveyed as electrical impulses, which are conducted by axons. Axons are part of neurones, extending from the cell body. The cell bodies of sensory neurones (afferent fibres) in spinal nerves are located in the dorsal root ganglia, while the cell bodies of the motor neurones (efferent fibres) are in the anterior horn of the spinal cord. The main metabolic activities of a neurone occur in the cell body, to which axons must stay connected in order to remain viable. There are antegrade and retrograde axonal transport mechanisms which pass chemical messages in both directions along axons.
Sensory neurones connect with the CNS via their central processes which pass through the dorsal roots to enter the spinal cord at the dorsal horn. They either synapse within the dorsal horn to neurones of the spinothalamic pathways or pass directly up the dorsal columns. Sensory information is thus passed to the sensory cortex and other parts of the brain. The importance of sensory information from the hand is reflected by a large area of sensory area of sensory cortex devoted to it. Motor neurones are activated by fibres of the cortico-spinal and other pathways which connect to them in the anterior horn of the spinal cord. Injury to a nerve has influences at all levels of the motor and sensory pathways both proximally and distally.
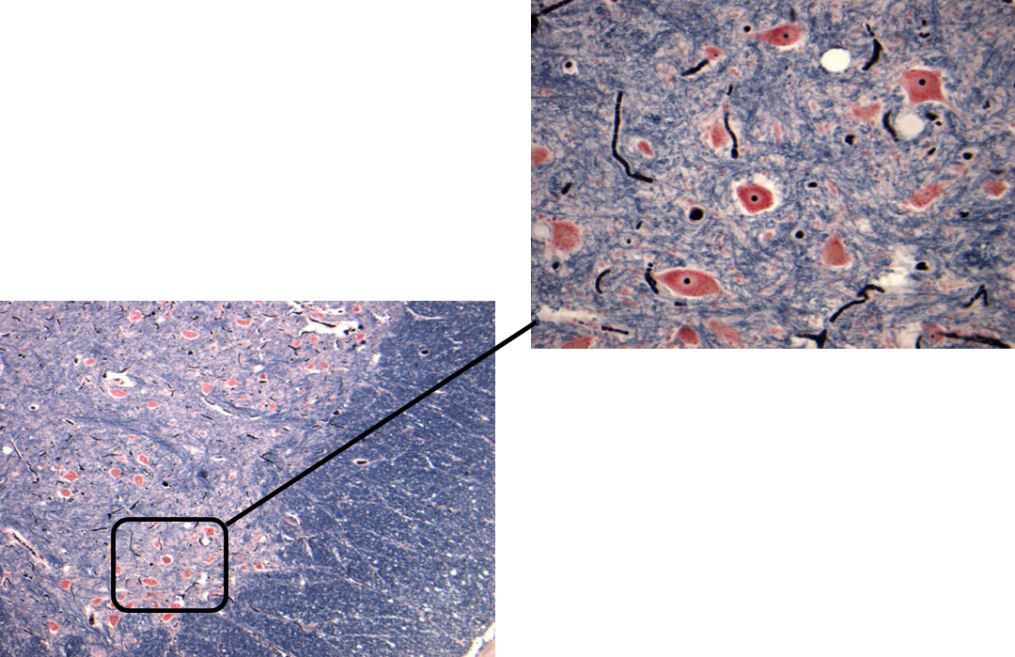
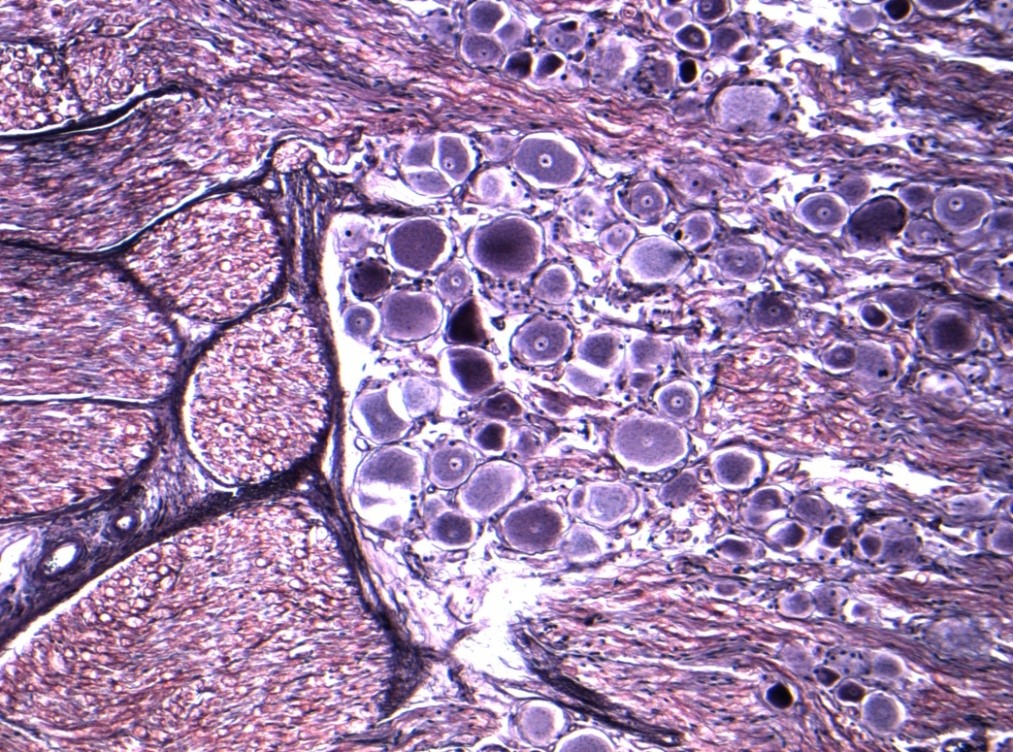
In peripheral nerves, axons are surrounded by Schwann cells, which form the insulating sheath around myelinated axons. A single Schwann cell wraps round a short length of the axon with the junctions between each cell being called nodes of Ranvier. Each Schwann cell is associated with a single myelinated axon. The combination of the axon and the myelin sheath surrounded by basement membrane or basal lamina is termed a nerve fibre. An intact myelin sheath is required for conduction of nerve impulses in myelinated fibres. A single Schwann cell wraps around several unmyelinated axons.
Normal axons have a resting membrane potential of –70 mV. An action potential is triggered by depolarisation of the membrane with the membrane potential shooting abruptly upwards to about +50 mV and then back downwards. The wave of depolarisation is propagated along the axon. The myelin sheath increases membrane resistance and allows faster, saltatory movement of action potentials from one node of Ranvier to the next.
The diameter of myelinated axons varies from 2 to 20 micrometres and unmyelinated axons from 0.1 to 1.25 micrometres. The conduction velocity in myelinated fibres is directly proportional to fibre diameter [2]. Gasser and Erlanger [3] classified nerve fibres on the basis of diameter and conduction velocity (see table 1).
Fibre type | Subtype | Function | Sensory end organs | |
---|---|---|---|---|
Group A | Largest myelinated fibres | Somatic afferent and efferent fibres | ||
A alpha | (15–20 micrometres) | Efferent motor fibres | ||
A beta | (8–15 micrometres) | Touch sensation | Rapidly and slowly adapting cutaneous mechanoreceptors (Meissner, Pacinian, Merkel and Ruffini corpuscles) | |
A delta | (2–5 micrometres) | Pain and temperature | Free nerve endings, thermoreceptors | |
Group B | Myelinated | Autonomic and preganglionic fibres | ||
Group C | Thinnest, unmyelinated (0.1–1 micrometres) | Visceral and somatic afferent (burning pain), postganglionic autonomic efferents | Free nerve ending nociceptors |
stained with Toluidine blue
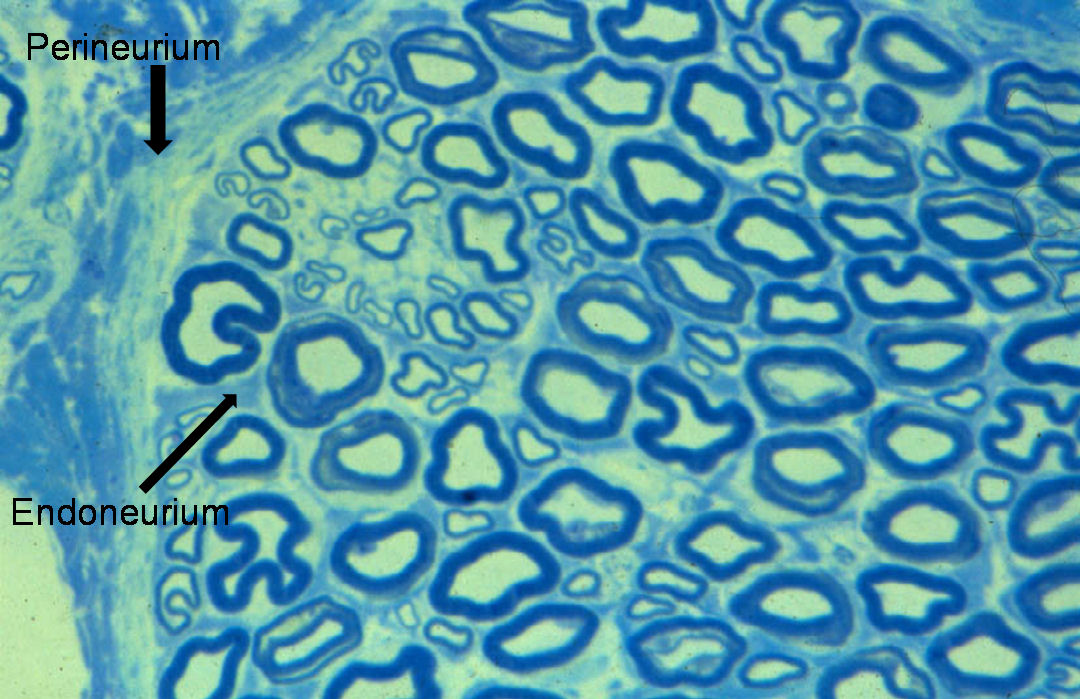
Nerve fibres are embedded in a loose connective tissue structure called Endoneurium, which consists of a collagenous matrix, fibroblasts, and a capillary network. Endoneurial collagen fibres and the Schwann cell basement membranes formendoneurial tubes which contain the nerve fibres. Large numbers of nerve fibres are grouped together into fascicles surrounded by a stronger sheath, the Perineurium. This acts as both a mechanical and diffusion barrier to maintain the internal environment of the endoneurium. The fascicles are bound together and the whole nerve trunk surrounded by another connective layer called the Epineurium. Fascicles undulate along the course of the nerve as do nerve fibres within the fascicles, thus allowing for some degree of stretch. The relative amount of epineurium varies along the course of nerves often being greater at joints and more distally. There is a vascular plexus within the epineurium with many longitudinal vascular channels. Hence nerves can usually be mobilised along a considerable length without causing significant ischaemia. Nerves are only very loosely attached to surrounding tissues therefore allowing considerable gliding associated with limb movement.
The arrangement of fascicles within nerve trunks was described in detailed work by Sunderland [4], in which he showed that they divide and join along the length of the nerve forming a plexus. The fascicular pattern doesn’t remain unchanged for more than 15 to 20 mm along the length of a nerve. Fibres with a particular function or destined for a specific branch are mixed within the fascicles in the proximal part of a nerve, but distally become located in a separate fascicle or fascicular bundle a few centimetres before the branch is given off. Hence accurate coaptation of a divided nerve is unlikely at a proximal site but more likely distally where fascicular bundles associated with each branch may be identifiable.
Nerve branches usually enter the deep surface of muscles nearer to their less mobile attachments (origins). After entering the muscle the nerve breaks up with fibres running in septa. The alpha motor fibres branch before terminating on a variable number of individual muscle fibres at the motor end plates. The muscle fibres innervated by a single alpha motor neuron are termed a motor unit. The size of motor units varies between muscles, with large units in proximal limb muscles and smaller units in muscles with precise action such as the intrinsic muscles of the hand. The muscle fibres innervated by one motor neuron are not necessarily grouped together, with considerable overlap between motor units.
Anatomy of nerves innervating the hand
Median nerve (C6, 7, 8, T1)
The median nerve is formed by contributions from the medial and lateral cords of the brachial plexus. It courses down the medial side of the arm closely related to the brachial artery lying first anterior and then medial to it by the time it reaches the elbow. On the anterior aspect of the elbow the nerve lies on the medial side of the antecubital fossa deep to the bicipital aponeurosis. It then passes into the forearm between the two heads of the pronator teres muscle and then deep to the fibrous arch of flexor digitorum superficialis (FDS). As it leaves the antecubital fossa it gives off branches including those to pronator teres, flexor carpi radialis (FCR), palmaris longus, and flexor digitorum superficialis (FDS), and the anterior interosseous nerve.
In the forearm the main trunk of the nerve runs distally on the deep surface of FDS emerging on the lateral side of the muscle a few centimetres proximal to the wrist. A further more distal branch is often given off to FDS of the index finger. The palmar sensory branch of the nerve is given off about 5cm proximal to the wrist and passes through the sheath of the FCR tendon just medial to the tendon at the level of the wrist crease. The anterior interosseous nerve runs more deeply between flexor digitorum profundus (FDP) and flexor pollicis longus (FPL). It supplies the radial half of FDP (index and middle fingers) and FPL before terminating in pronator quadratus which it also supplies.
At the wrist the nerve passes through the carpal canal where it lies superficial (anterior) to the flexor tendons of the fingers. At the distal end of the carpal canal the nerve divides into its terminal branches. These include the thenar motor branch, which curves back over the distal edge of the flexor retinaculum to enter the thenar muscle which it supplies. The thenar motor branch occasionally penetrates the flexor retinaculum more proximally. There may be a contribution from the deep motor branch of the ulnar nerve to innervation of the opponens pollicis and flexor pollicis brevis. The abductor pollicis brevis is consistently supplied by the median nerve. There are also motor branches to the radial two lumbricals. The remaining branches are sensory with usual four initial branches, one to the radial side of the thumb, a common nerve later divides into the digital nerves on the ulnar side of the thumb and the radial side of the index finger, and then common digital nerves to adjacent sides of the index and middle, and middle and ring fingers. These sensory branches run distally coming to lie on either side of the flexor sheaths in each digit (Figure 7a). The median nerve provides sensory innervation to much of the palm of the hand and the palmar aspects of the thumb, index, middle and radial half of the ring finger, as well as the dorsum of the fingers around the nails. Fibres originate from C6, C7, and C8 (Figure 7b), although it should be noted that there is considerable overlap of dermatome areas.
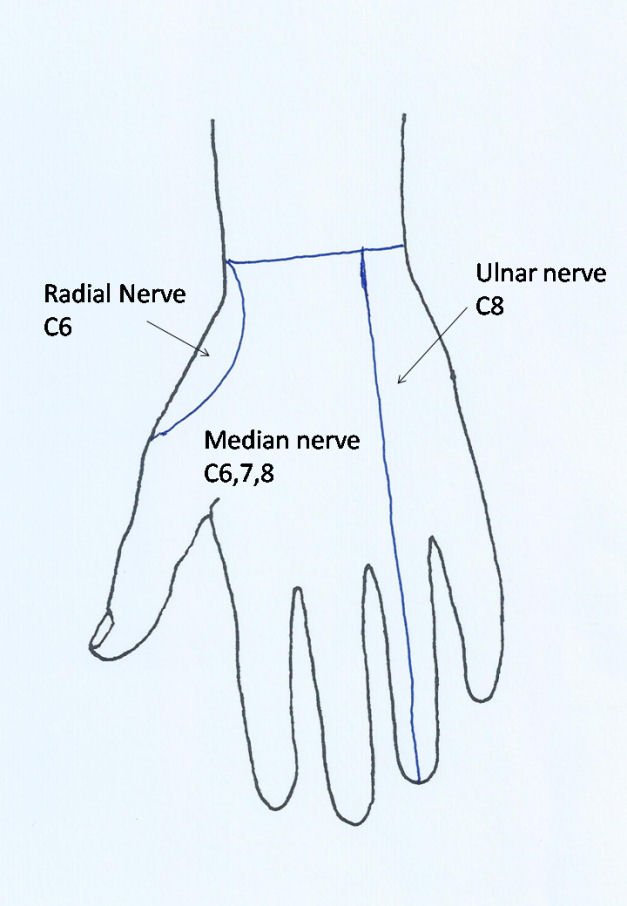
The root innervation values for the muscles of the forearm and the hand (myotomes) are listed in table 2. Observation of the effects of brachial plexus injuries (Bell and Hems, In press [5]) indicate that there is considerable innervation from the T1 root to the median nerve innervated muscles including FDS, the radial half of FDP, FPL, palmaris longus, and the thenar muscles. The C7 input to the radial finger flexors and FPL is variable with these often being deficient in lesions of the lower trunk of the brachial plexus. The pronator teres and FCR are fairly consistently innervated from C6 and C7.
Muscle | Myotome |
---|---|
Supraspinatus | C5 C6 |
Infraspinatus | C5 C6 |
Deltoid | C5 C6 |
Pectoralis major |
C5 C6 C7 C8 |
Latissimus dorsi | C6 C7 C8 |
Biceps | C5 C6 (C7) |
Triceps | C6 C7 C8 |
Wrist Extensor (Radial) | C6 C7 |
EDC | C7 C8 T1 |
EPL | C7 C8 T1 |
FCR | C6 C7 |
FCU | C7 C8 |
FDP Radial Digits | C8 T1 |
FDP Ulnar Digits | C7 C8 T1 |
FDS | C8 T1 |
FPL | C7 C8 T1 |
Thenar | C8 T1 |
Interossei | C7 C8 T1 |
Ulnar nerve (C7, 8, T1)
The ulnar nerve is one of the terminal branches of the medial cord of the brachial plexus. It runs down the medial side of the arm posterior to the brachial artery and then penetrates the medial intermuscular septum at about the mid humerus level to lie on the medial side of the triceps muscle. There are usually no branches above the elbow but occasionally it gives a small branch to the medial head of triceps [7].
At the elbow it runs behind the medial epicondyle in the cubital tunnel before passing between the humeral and ulnar heads of flexor carpi ulnaris (FCU). As well as articular branches to the elbow it gives off branches to the FCU soon after entering the muscle. In the forearm it runs distally between FCU and FDP adjacent to the ulnar artery in the distal two thirds. Further branches are given off to FCU and to the medial half of FDP. These typically innervate flexion of the ring and little fingers although there is overlap with the median nerve with regard to the middle finger. About 5 cm proximal to the wrist crease the ulnar nerve gives off a dorsal sensory branch which runs posterior to the tendon of FCU to reach the ulnar border of the wrist passing over the ulnar styloid before branching over the dorsum of the hand to supply sensation to the dorsum of the ring and little fingers. The dorsal sensory branch is vulnerable to damage during operations on the distal ulna.
At the wrist the nerve enters Guyon’s canal lying lateral to the pisiform and superficial to the pisohamate ligament. As it emerges from the canal it divides typically into a superficial branch, which continues distally to provide sensation to the little finger and ulnar side of the ring finger, and a deep motor branch. There are also one or branches to abductor digiti minimi. Gudemez et al. [8] described the pattern of branching and its variants.
The deep motor branch curves round the hook of the hamate and under opponens digiti minimi and then runs laterally across the front of the interosseous muscles which it supplies as well as the medial two lumbricals. It terminates in the adductor pollicis. It may also give branches to the thenar muscles (see median nerve above).
The ulnar nerve receives fibres from the C7, C8, and T1 nerve roots (see table 2). Although variable, the contribution from C7 is often significant with patients, who have suffered injury to the lower trunk of the brachial plexus, retaining function in FCU and the FDPs to the ring and little fingers. There may also be some innervation to the intrinsic muscles of the hand particularly adductor pollicis. The C8 root appears to make the largest contribution to the ulnar nerve with lesions to this root producing weakness and flexion of the little finger and partial paralysis of the intrinsic muscles to the hand. The sensory innervation is also largely within the C8 dermatome.
Radial nerve (C6, 7, 8, T1)
The radial nerve is formed as one of the terminal branches of the posterior cord of the brachial plexus. It runs distally posterior to the brachial artery and then passes through the triangular space formed by the tendons of teres major and latissimus dorsi, the humeral shaft, and the long head of triceps to reach the spiral groove. Its course then spirals distally around the posterior aspect of the mid shaft of the humerus to pass through the lateral intermuscular septum at the junction of the distal and middle thirds of the arm. As the nerve enters the spiral groove it gives off branches to the triceps, the first being to the long head of triceps and then to the lateral and medial heads. That to the medial head is the thinnest and longest passing on down distal to the elbow to supply anconeus. The posterior cutaneous nerve of the forearm also branches at this level and penetrates the lateral head of triceps to descend on the lateral side of the arm and the dorsum of the forearm supplying skin on its course.
In the distal arm the radial nerve runs between the brachialis muscle and brachioradialis. It gives branches to the lateral third of the brachialis and to brachioradialis and extensor carpi radialis longus (ECRL). At the level of the elbow it divides into the superficial radial nerve and posterior interosseous nerve. The superficial radial nerve runs down the forearm on the deep surface of brachioradialis, closely associated with the radial artery in the middle third of its course, before passing backwards under the tendon of brachioradialis to become subcutaneous on the radial aspect of the wrist. It is closely related to the radial styloid where it is vulnerable to injury from lacerations or surgery. It then branches over the dorsum of the hand to provide sensation on the back of the thumb, index and middle fingers (Figure 7c).
The posterior interosseous nerve gives a branch to extensor carpi radialis brevis (ECRB) before passing backwards on the lateral aspect of the proximal radius between the deep and superficial heads of the supinator muscle which it supplies. It then emerges from the supinator on the dorsum of the forearm at the junction of its middle and distal thirds. At that level it immediately gives branches which curve superficially to supply the overlying extensor digitorum communis (EDC). The nerve then divides out into its terminal branches which innervate the remaining muscles of the extensor compartment of the forearm. The pattern of branching has been described in detail by Branovacki et al [9]. Typically the branch to the extensor pollicis longus is the most distal motor branch. A slender branch continues distally along the interosseous membrane and then deep to the fourth dorsal tendon compartment to reach the wrist joint which it also innervates.
The radial nerve receives fibres from the C6 through to T1 roots. The triceps receives fibres from C6, C7, and C8, with typically good power remaining even if two of these roots have been damaged by injury to the brachial plexus. The brachioradialis is innervated largely from C6, with the radial wrist extensors being innervated from C6 and C7 and from extensor carpi ulnaris (ECU) by C7 and C8. The main innervation to the finger and thumb extensors is from C7 and C8. The contribution from C7 is variable but more dominant in the extensors of the ulnar digits. There is a small contribution from T1 which is more significant in the thumb extensors and extensor indicis [5].
Response of nerve to injury
Peripheral nerves may be damaged by blunt trauma, such as compression or stretch, or by sharp injury causing laceration. Compression may be acute or chronic. The pathology of chronic compression of nerve will be described in more detail in the section on compression neuropathy.
Conduction block
Mild compression, stretch or ischaemia of a nerve may cause a conduction block. In this type of injury, the axonal and overall structure of nerve fibres is preserved. The abnormality is confined to the affected segment of the nerve. While conduction is blocked over the affected segment it remains present in both the proximal and distal segments of the nerve. Because this type of injury recovers spontaneously human material for histological examination is rarely available. Therefore the evidence on pathology is largely dependent on experimental studies. Based on the duration of conduction block, Sunderland [10] described three grades of severity:
- Transient conduction block, which lasts only a few minutes or hours, and is thought to be related to temporary ischaemia of the nerve.
- A moderately severe lesion occurs when the conduction block lasts for up to about 4 weeks. The injured section of nerve is oedematous, hyperaemic and infiltrated with macrophages and lymphocytes. There are abnormalities in the myelin sheaths particularly adjacent to the nodes of Ranvier. Myelin sheaths may be thinned.
- In severe conduction block lesion, which lasts for several months, there is thinning of axons and localised demyelination. There is also telescoping of myelin at the nodes, which is seen particularly at the margins of the compressed nerve segment.
In all types of conduction block the large diameter myelinated fibres are more vulnerable. Motor fibres are generally more susceptible than sensory fibres. Providing the compression is relieved the changes in conduction block lesions are fully reversible so that complete recovery occurs. Ischaemia appears to be an important element in causing conduction block lesion. Compression of a nerve inevitably causes some degree of ischaemia. If ischaemic lasts for more than about 6 hours then axons will be damaged to an extent that axonal degeneration will occur.
Axonal degeneration
If an injury to a nerve is sufficient to disrupt the axons then the process of Wallerian degeneration ensues in the nerve below the site of the injury. Axons may be disrupted alone, with the connective tissue layers of the nerve intact, usually as a result of prolonged compression, or the whole nerve trunk may be divided (see later section for further details). If axons are divided then the part of the axon below the injury is disconnected from its cell body. Within 2 to 4 days the axon ceases to conduct and then fragments. Within a few days myelin sheaths also fragment. There is proliferation of Schwann cells. Macrophages migrate into the endoneurial tubes and together with the Schwann cells phagocytose the myelin debris and axons (see histological section, figures 8 and 9). The distal segment of nerve is then left with endoneurial tubes containing Schwann cells. The endoneurial tubes gradually shrink and there is an increase in endoneurial collagen (see figures).
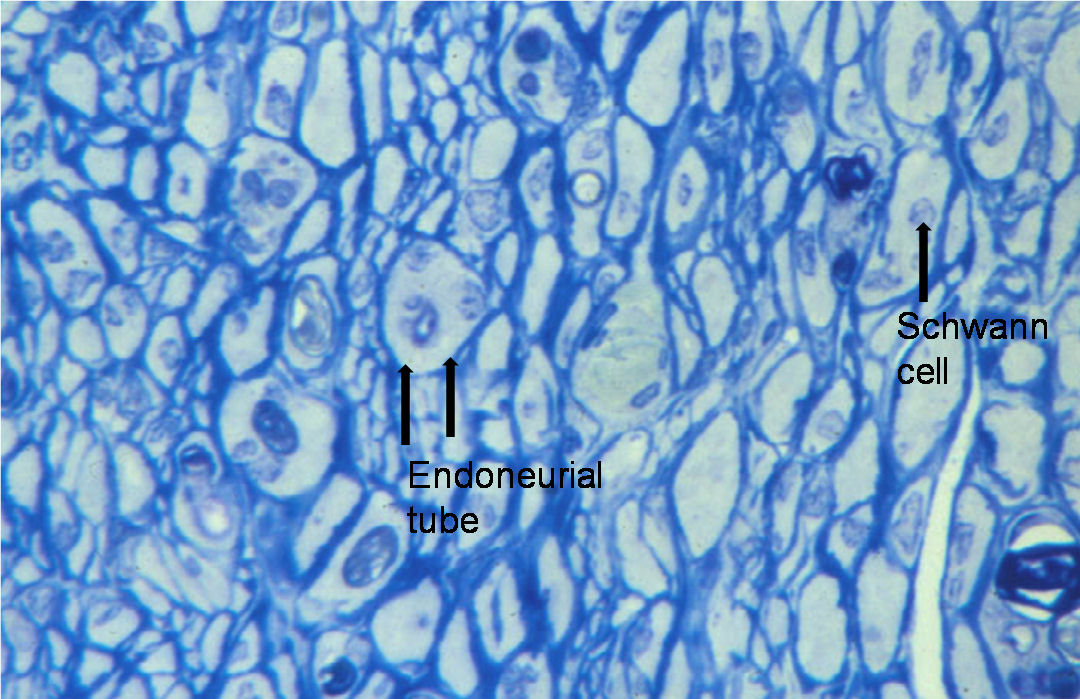
There is some degree of degeneration of axons above the level of the injury. The extent of this retrograde degeneration appears to be related to the severity of the injury. It is greater when a nerve is ruptured by violent stretch injury than when it is cleanly transected. It is also greater in more proximal injuries to nerves.
In addition to retrograde degeneration of axons some distance above the injury site there are metabolic changes in the cell bodies. Some neurons degenerate and are lost, while others enter a recovery phase. There is therefore a reduction in the number of neurons after an injury [11]. It appears that myelinated sensory fibres are more severely affected than motor fibres.
Rapid changes occur in denervated muscle with loss of weight and atrophy of fibres. About 30% of the weight is lost in the first month reaching over 60% loss by the 4th month [12]. There is loss of sarcoplasm and reduction in calibre of muscle fibres (see figure 10). These changes account for the wasting of denervated muscle which is observed on clinical examination. The amount of connective tissue in the muscle increases with widespread interstitial fibrosis after long term denervation. While muscle tissue can survive denervation for at least 2 years, in the longer term irreversible degenerative changes occur with thinning and obliteration of fibres and replacement with strands of fibrous tissue and fat cells.
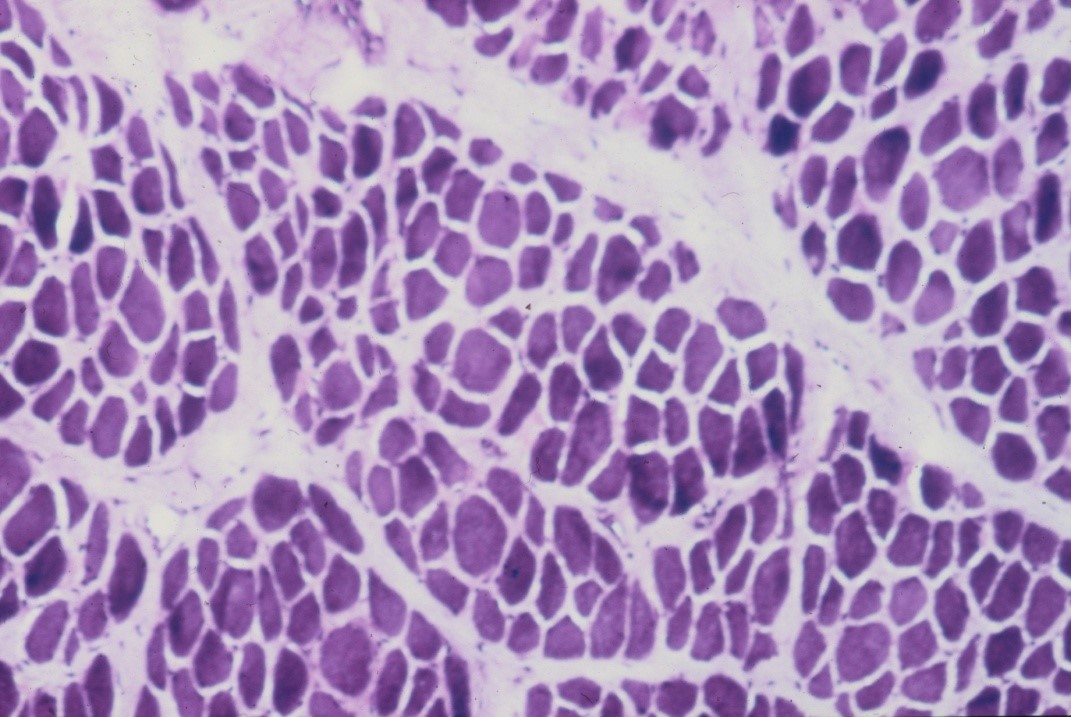
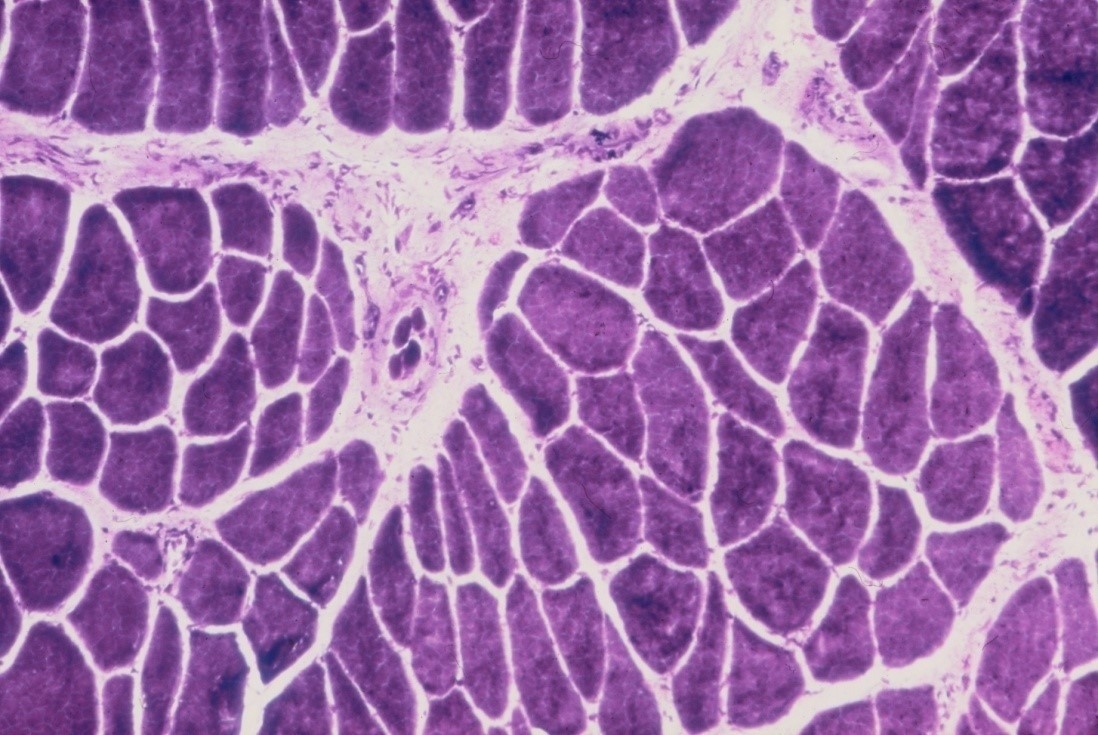
Axon regeneration
Providing the nerve cell survives the axon may sprout and regenerate distally. This process starts within a few days of injury or may be delayed in more severe injuries. The axons then grow distally to the site of injury. Axonal regeneration and indeed survival of the neurons depends on a variety of growth factors. The proliferating Schwann cells in the distal segment of the nerve appear to be important in producing these substances. If the connective tissue of the nerve is intact progress on to the distal segment is uncomplicated with axons following the same endoneurial tube which they previously occupied. If the ends of a divided nerve are approximated then the regenerating axons may be able to reach the endoneurial tubes in the distal nerve. Axons may regenerate across a short (1–2 cm) distance between the ends of a divided nerve. However, the quality of regeneration is inferior to that after good nerve suture. If a divided nerve is not repaired, then the fibres attempting to regenerate from the proximal stump will form a neuroma.
Because of the large numbers of fibres in a nerve trunk there will be disruption and mixing of fibres at the site of the repair. The axons grow distally. Schwann cells become aligned along them and then reform the myelin sheath. Regeneration is slow being only 1 to 2 mm per day in humans. The regenerating axons eventually reconnect with muscles and sense organs. Nerves that have regenerated after axonal degeneration remain abnormal. The fibre diameter is reduced and hence conduction velocity [13] (see figure 11). There is increased endoneurial fibrosis.
As mentioned above there is mixing of regenerating axons if the connective tissue layers of the nerve have been disrupted. This raises the question as to whether there are any biological factors which favour specificity of regeneration. There is tissue specificity with regenerating axons growing preferentially into nerve tissue. There is some evidence for motor-sensory specificity with preferential motor axonal reinnervation of motor targets and corresponding preferential sensory axonal reinnervation of sensory targets [14], [15], [16]. This is at least partly achieved by selective loss of axon sprouts which have regenerated along an incorrect pathway. Topographic specificity is the preferential re-establishment of axonal pathways into corresponding topographical subcomponents of a nerve trunk. There appear to be no biological factors which confer topographic specificity. Therefore matching nerve ends during nerve repair is the only measure which can improve topographic specificity and this is, at best, only at the level of fascicles.
When regenerating axons connect with muscle fibres there is a reversal of the changes in the muscle which occur on denervation, with increasing fibre diameters and muscle bulk (see figure 10c). As a result of neuronal loss there is a reduced number of axons re-innervating a muscle resulting in the formation of fewer but larger motor units. If there is a partial injury to a motor nerve where some axons degenerate, then partial denervation of innervated muscles will occur. Weakness of the muscles will not usually be apparent clinically until approximately 50% of the motor axons are lost. Remaining axons will subsequently branch within the muscle in order to reinnervate denervated muscle fibres, thereby forming larger motor units. Muscle strength will improve even though damaged axons have not actually regenerated.
References
[1] Tubiana R, Thomine J-M, Mackin E. Examination of the Hand and Wrist. London: Martin Dunitz; 1996.[2] Rushton WA. A theory of the effects of fibre size in medullated nerve. J Physiol. 1951 Sep;115(1):101-22. DOI: 10.1113/jphysiol.1951.sp004655
[3] Gasser HS, Erlanger J. The role played by the sizes of the constituent fibers of a nerve trunk in determining the form of its action potential wave. Am J Physiol. 1927;80:522-47. DOI: 10.1152/ajplegacy.1927.80.3.522
[4] Sunderland S. Nerves and Nerve Injuries. 2nd ed. Edinburgh: Churchill Livingstone; 1978.
[5] Bell SW, Brown MJ, Hems TJ. Refinement of myotome values in the upper limb: Evidence from brachial plexus injuries. Surgeon. 2017 Feb;15(1):1-6. DOI: 10.1016/j.surge.2015.08.004
[6] O'Brien M. Aids to the examination of the peripheral nervous system. 5th ed. Oxford: Elsevier; 2010.
[7] Miguel-Pérez MI, Combalia A, Arandes JM. Abnormal innervation of the triceps brachii muscle by the ulnar nerve. J Hand Surg Eur Vol. 2010 Jun;35(5):430-1. DOI: 10.1177/1753193409346909
[8] Gudemez E, Tekdemir I, Uslu M, Eksioglu F, Elhan A. Innervation pattern of the abductor digiti minimi muscle of the hand. J Hand Surg Br. 2002 Oct;27(5):435-9. DOI: 10.1054/jhsb.2002.0784
[9] Branovacki G, Hanson M, Cash R, Gonzalez M. The innervation pattern of the radial nerve at the elbow and in the forearm. J Hand Surg Br. 1998 Apr;23(2):167-9. DOI: 10.1016/S0266-7681(98)80166-6
[10] Sunderland S. Nerve Injuries and their Repair. A critical appraisal. Edinburgh: Churchill Livingstone; 1991.
[11] McKay Hart A, Brannstrom T, Wiberg M, Terenghi G. Primary sensory neurons and satellite cells after peripheral axotomy in the adult rat: timecourse of cell death and elimination. Exp Brain Res. 2002 Feb;142(3):308-18. DOI: 10.1007/s00221-001-0929-0
[12] Sunderland S, Ray LJ. Denervation changes in mammalian striated muscle. J Neurol Neurosurg Psychiatry. 1950 Aug;13(3):159-77. DOI: 10.1136/jnnp.13.3.159
[13] Cragg BG, Thomas PK. The Conduction velocity of regenerated peripheral nerve fibres. J Physiol. 1964 May;171:164-75. DOI: 10.1113/jphysiol.1964.sp007369
[14] Brushart TM, Seiler WA 4th. Selective reinnervation of distal motor stumps by peripheral motor axons. Exp Neurol. 1987 Aug;97(2):289-300. DOI: 10.1016/0014-4886(87)90090-2
[15] Brushart TM. Preferential motor reinnervation: a sequential double-labeling study. Restor Neurol Neurosci. 1990 Jan;1(3):281-7. DOI: 10.3233/RNN-1990-13416
[16] Rath S, Green CJ. Selectivity of distal reinnervation of regenerating mixed motor and sensory nerve fibres across muscle grafts in rats. Br J Plast Surg. 1991;44:215-218. DOI: 10.1016/0007-1226(91)90130-C