Identification of influence factors on the quality and shelf life of fresh meat throughout the supply chain
Lucas Correa Dresch 1
1 Institute of Animal Sciences, University of Bonn, Bonn, Deutschland
Abstract
Due to the industrialization and globalization of food production, food supply chains became increasingly complex, which led to a multitude of steps that products must pass during production, processing and distribution. At the same time, the quality standards and requirements for shelf life were raised in order to allow for the most efficient delivery and exploitation of the sales period of fresh products, especially for meat. During the supply, meat is exposed to several environments from farm to fork which might affect the quality and shelf life of the good. This work aims at the identification of the most important influence factors based on a literature review. First, the quality connotation of meat is defined and different aspects of meat quality are analyzed. Second, freshness and the spoilage process of fresh meat are characterized. Then, four main categories of factors influencing quality and shelf life of meatare identified, namely animal-specific, product-specific, process-specific and environmental factors. The impact of these factors is discussed by means of examples as well as graphs based on the literature review. The results are summed up in the conclusion leading to an outlook which points to future research prospects and the actual lack of investigations.
Introduction
The supply chain of fresh food is comprised of a complex set of processes that ensure quality and safety of the products, and at the same time maintain a sustainable, environmentally friendly production
As the food market outgrew local markets, the food industry shifted from single protagonists to supply chains; organizations, practitioners, consultants, and academics recognized that only enhancing performance throughout in-house practices within their industry is not sufficient
Another important requirement for the meat industry is an environmentally friendly and sustainable production
This research intends to analyze and clarify the parameters that influence quality and shelf life of fresh meat products throughout the supply chain. First, meat quality will be defined and the characteristics and influences on the spoilage process of meat are elucidated. Next, it will present and define important parameters that should be considered when managing quality and safety of meat through the supply chain, from farm to fork, while elucidating at the same time their influence on production, marketability, and overall food waste.
Quality parameters and the spoilage process of fresh meat
A high quality of fresh food is crucial for the subsequent processing, stability, and salability of the products. With an increasing complexity of production and supply chains, the persistence of food quality and suitability for storage is an important requirement for every actor in the chain. As quality is one major driver for the purchase decision, there is an increasing sensitivity in the food production industry that competing on price alone is not sufficient to satisfy the refining consumer demands
The quality of food is defined as
"the sum of value-determining properties of food, which define the degree of utilization for the prescribed purpose"
Compared to other fresh products, meat is a product that is characterized by a particular complexity. The term meat quality comprises a set of different inherent characteristics. Meat quality is defined by the compositional quality, the functional quality, and the palatability
Since fresh meat is no stable product but undergoes different biological, physicochemical and microbial activities, meat quality is a dynamic state which is continuously moving to reduced levels
In general,
"spoilage of food involves any change which renders food unacceptable for human consumption and may result from a variety of causes"
Spoilage can have several causes, such as microbial growth and metabolism, insect harm, physical damage, the activity of intrinsic enzymes as well as chemical processes. For fresh meat, most quality changes during spoilage are initiated by three main mechanisms
Fresh meat is distinguished by a high water content, a large amount of nutrients, and an optimal pH-value for the growth of microorganisms. The nutritional value may vary between different meat types, but is generally constituted by the main component water (70%), followed by protein (20%), lipids (<10%) and ash (1%)
![]() |
The shelf life of meat or meat products is described as the time of storage until the product is spoiled. The point of spoilage is defined as
"a certain maximum acceptable bacterial level, or an unacceptable off-odor/off-flavor or appearance. The shelf-life depends on the numbers and types of microorganisms, mainly bacteria, initially present and their subsequent growth"
During slaughter and processing, fresh meat is contaminated with microorganisms emerging from animal microbiota as well as microorganisms of human or environmental origin. The bacteria are transferred to the product via contaminated machines, surfaces and the aerosols in the slaughterhouse
By knowing a few physical and chemical properties of the food, the prediction of the SSO and their growth is possible
Influence factors on meat quality and shelf life
The factors influencing meat quality and shelf life can be subdivided into four major categories. These factors comprise the complete value-added chain, from the animal production to consumption. Each of the four categories can be assigned to one essential element in the production or storage process, including also the properties of the product itself. The influence factors on meat quality and shelf life are subdivided into the animal-specific factors, product-specific factors, process-specific factors and environmental factors (figure 1).
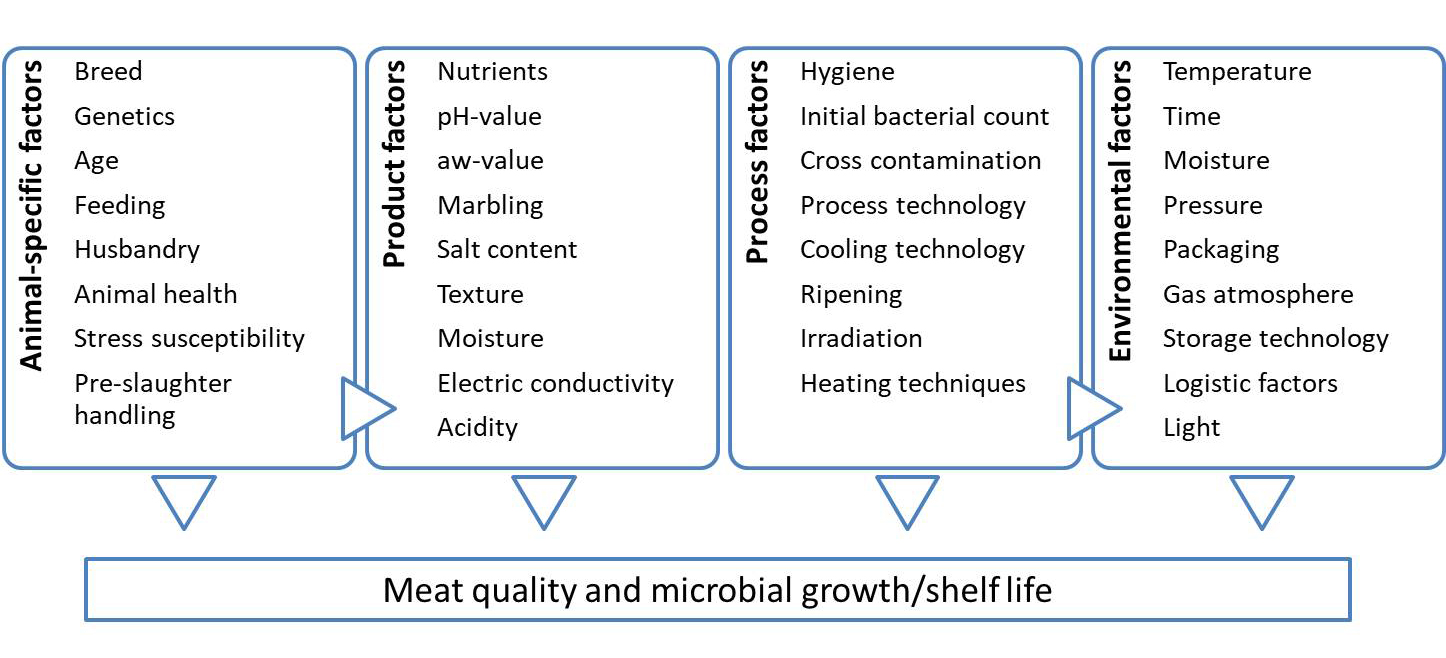
The animal-specific factors focus on the first steps in the meat production. Even though the effects of genetic selection and adjusted diets are well-investigated, a comprehensive view on meat quality and shelf life from farm to fork is often not considered. The choice of breed has a crucial impact on the meat composition, the fat and protein content, and, as a result, the meat quality, as well as nutritional value
Next to pre-slaughter stress, the diet has a noticeable impact on the color and color stability of meat
The addition of essential amino acids in broiler diet, such as methionine or lysine, enhances performance parameters, meat yield and final body weight. Furthermore, the diet also regulates the final pH, drip loss and color of poultry meat
The adjustment of the diet is often accompanied by a changed growth velocity and performance of the animals, which is also considered during the development of alternative husbandry systems. Organic production systems with outdoor access, enhanced roaming, adjusted nutrition and the targeted choice of slow growing races result in significant differences in certain meat quality parameters, compared to the conventional industrial meat production
The product-specific factors comprise all intrinsic properties which are typical for fresh meat. The meat composition and the nutritional value influence the storage stability through the availability of nutrients and key substrates, such as glucose
The pH-value is one further product-specific factor with major importance for the growth rate of microorganisms
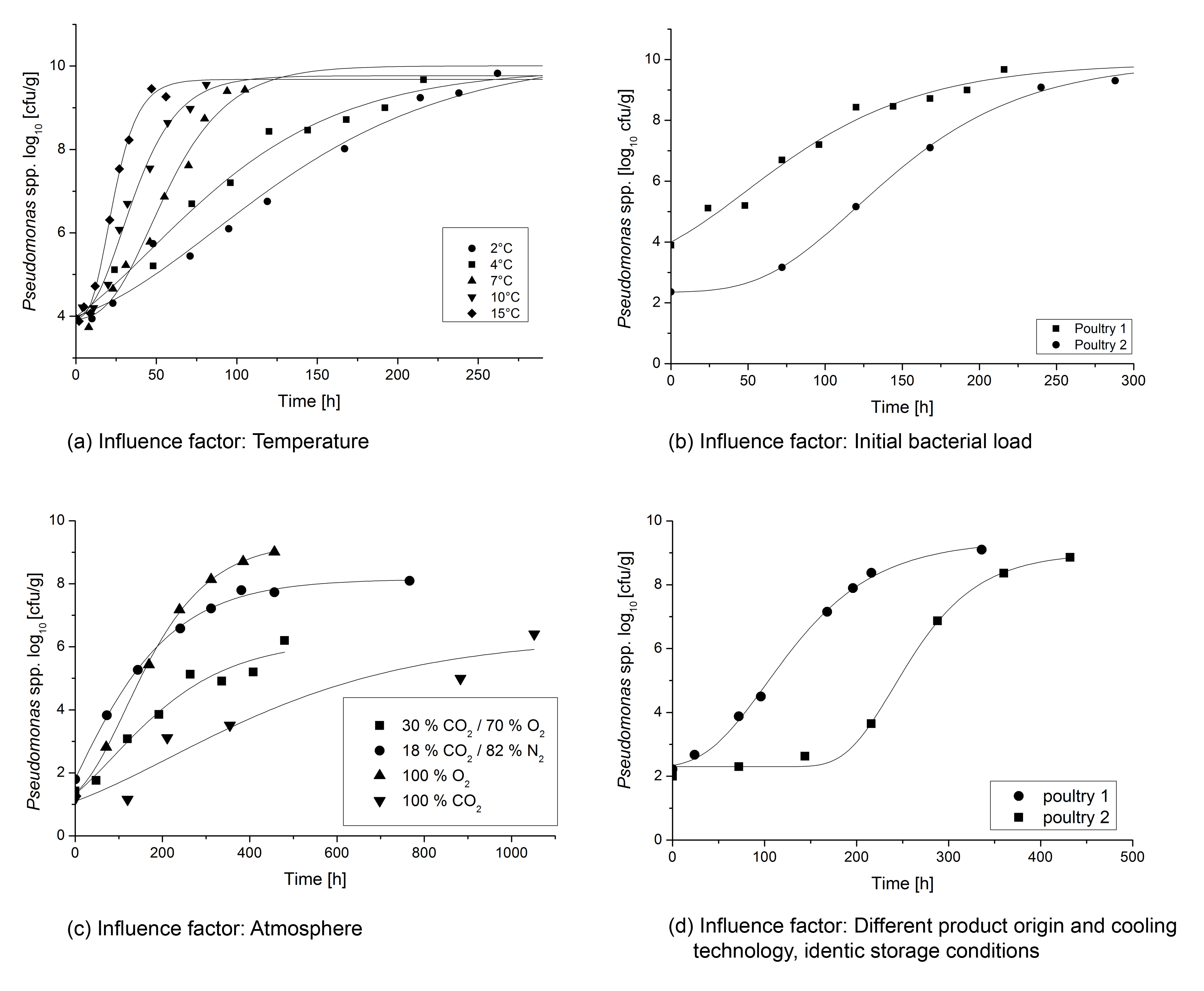
The process-specific factors include influence factors within the slaughter and processing facilities. The education of employees, industrial hygiene, equipment, and the cleaning routines significantly affect the initial contamination of the product
For the length of shelf life, the environmental factors are of major importance. They are determined by a set of different drivers referring mostly to the storage conditions of the meat. Intensive research efforts focused on the impact of environmental factors on fresh meat in order to control the cold chain and prolong the shelf life with advanced packaging technologies. The temperature is supposed to have the highest influence on the stability of meat products (figure 2.a). Due to its ability to accelerate microbial growth and metabolism as well as biochemical and physical processes, temperature has a crucial impact on the quality, safety and shelf life of meat
For fresh poultry stored at 4°C, the shelf life can be prolonged from 100h (aerob) to 212h by using a 70% O2-packaging
Conclusion
As the meat market reached the saturation point in western countries, the requirements from consumers regarding high quality and sustainable products increased
Additionally, post-production quality control alone is not sufficient for a food company to stand out on the market as the quality control of fresh meat products requires the facilitation of real-time monitoring processes and intensive communication of relevant information between members of the supply chain
Thus, quality and safety schemes for meat products can be adjusted accordingly for a more effective control throughout the entire chain.
References
[1] Dohlen S, Albrecht A, Kreyenschmidt J. Sustainable packaging solutions to improve resource efficiency in supply chains of perishable products. In: Kreyenschmidt J, Dohlen S, editors. Living Handbook of Perishable Food Supply Chains. Cologne: PUBLISSO; 2016. DOI: 10.5680/lhpfsc000002[2] Troy DJ, Ojha KS, Kerry JP, Tiwari BK. Sustainable and consumer-friendly emerging technologies for application within the meat industry: an overview. Meat Sci. 2016;120:2-9. DOI: 10.1016/j.meatsci.2016.04.002
[3] Pozo H, França Barcelos A, Kazue Akabane G. Critical factors of success for quality and food safety management: classification and prioprization. UJIBM. 2018;6(2):30-41. DOI: 10.13189/ujibm.2018.060202
[4] Lehmann RJ, Reiche R, Fritz M, Schiefer G, editors. Information services for agri-food supply chains – a framework for development. In: 31 GIL-Jahrestagung, 2011 Feb 24-25; Oppenheim, Germany. Available from: https://gil-net.de/Publikationen/23_125.pdf
[5] Maldonado E, Zaragoza-Ramírez JL, Martínez-Hernández PA, González-Ariceaga CC, García-Méndez JL. Cold chain conditions of meat and dairy products in mexican retail food stores. In: Kreyenschmidt J, Dohlen S, editors. Living Handbook of Perishable Food Supply Chains. Cologne: PUBLISSO; 2016. DOI: 10.5680/lhpfsc000001
[6] Schiefer G. Environmental control for process improvement and process efficiency in supply chain management - the case of the meat chain. Int J Prod Econ. July 2002;78(2):197-206. DOI: 10.1016/S0925-5273(01)00166-9
[7] Schulze-Ehlers B, Anders S. Towards consumer-driven meat supply chains: opportunities and challenges for differentiation by taste. Renew Agric Food Syst. 2018;33(1):73-85. DOI: 10.1017/S1742170517000059
[8] Siddh MM, Soni G, Jain R, Sharma MK. Structural model of perishable food supply chain quality (PFSCQ) to improve sustainable organizational performance. Benchmarking. 2018;25(7):2272-317. DOI: 10.1108/BIJ-01-2017-0003
[9] Raab V, Petersen B, Kreyenschmidt J. Temperature monitoring in meat supply chains. BFJ. 2011;113(10):1267-89. DOI: 10.1108/00070701111177683
[10] Juan Ding M, Jie F, Parton KA, Matanda MJ. Relationships between quality of information sharing and supply chain food quality in the Australian beef processing industry. IJLM. 2014;25(1):85-108. DOI: 10.1108/IJLM-07-2012-0057
[11] Guochen W. Research on Fresh Meat Cold Chain Logistics Service Quality Model based on supply chain. JIM. 2016;5(3):17-24. DOI: 10.11648/j.jim.20160503.11
[12] Grunert KG. Food quality and safety: consumer perception and demand. Eur Rev Agric Econ. 2005;32(3):369-91. DOI: 10.1093/eurrag/jbi011
[13] Hammes WP. Lebensmittelkunde. In: Biesalski HK, Fürst P, Kasper H, Kluthe R, Pölert W, Puchstein C, Stähelin H, editors. Ernährungsmedizin. Stuttgart: Thieme; 2004.
[14] ElMasry G, Sun DW. Meat quality assessment using a hyperspectral imaging system. In: Sun DW, editor. Hyperspectral imaging for food qualtity analysis and control. Elsevier; 2010. p. 175-240. DOI: 10.1016/B978-0-12-374753-2.10006-1
[15] FAO. FAO’s animal production and health division: meat & meat products [Internet]. c2019 [cited 2021 March 1]. Available from: http://www.fao.org/ag/againfo/themes/en/meat/home.html
[16] Grunert KG, Bredahl L, Brunsø K. Consumer perception of meat quality and implications for product development in the meat sector – a review. Meat Sci. 2004;66(2):259-72. DOI: 10.1016/S0309-1740(03)00130-X
[17] Verbeke WAJ, Viaene J. Ethical challenges for livestock production: meeting consumer concerns about meat safety and animal welfare. J Agric Environ Ethics. 2000;12:141-51.
[18] Font-I-Furnols M, Guerrero L. Consumer preference, behavior and perception about meat and meat products: an overview. Meat Sci. 2014;98(3):361-71. DOI: 10.1016/j.meatsci.2014.06.025
[19] Grunert KG, Sonntag WI, Glanz-Chanos V, Forum S. Consumer interest in environmental impact, safety, health and animal welfare aspects of modern pig production: results of a cross-national choice experiment. Meat Sci. 2018;137:123-9. DOI: 10.1016/j.meatsci.2017.11.022
[20] Taoukis PS, Labuza TP, Saguy IS. Kinetics of food deteriotation and shelf-life prediction. In: Valentas KJ, Rotstein E, Singh RP, editors. Handbook of food engineering practice. Boca Raton, Fla: CRC; 1997. p. 366-408.
[21] Bruckner S, Albrecht A, Petersen B, Kreyenschmidt J. A predictive shelf life model as a tool for the improvement of quality management in pork and poultry chains. Food Control. 2013;29(2):451-60. DOI: 10.1016/j.foodcont.2012.05.048
[22] Forsythe SJ, Hayes PR, editors. Food hygiene, microbiology and HACCP. Boston, MA: Springer US; 1995.
[23] Dave D, Ghaly AE. Meat spoilage mechanisms and preservation techniques: a critical review. Am J Agric Biol Sci. 2011;6:486-510.
[24] Huis in’t Veld JHJ. Microbial and biochemical spoilage of foods: an overview. Int J Food Microbiol. 1996;33(1):1-18. DOI: 10.1016/0168-1605(96)01139-7
[25] Lambert AD, Smith JP, Dodds KL. Shelf life extension and microbiological safety of fresh meat – a review. Food Microbiol. 1991;8:267-97.
[26] Krämer J. Lebensmittel-Mikrobiologie. Stuttgart: UTB; 2002.
[27] Belitz HD, Grosch W, Schieberle P. Food chemistry. Berlin, Heidelberg: Springer; 2009.
[28] Reuter G. Mikrobiologie des Fleisches. In: Weber H, editor. Fleisch – Fisch – Feinkost. Hamburg: Behr; 2003. p. 112.
[29] Nychas GJE, Dillon VM, Board RG. Glucose, the key substrate in the microbiological changes occurring in meat and certain meat products. Biotechnol Appl Biochem. 1988 Jun;10:203-31.
[30] Nychas GJE, Marshall DL, Sofos JN. Meat, poultry and seafood. In: Beuchat LR, Doyle MP, editors. Food microbiology: fundamentals and frontiers. 3rd ed. Washington: American Society of Microbiology; 2007. p. 105-40. DOI: 10.1128/9781555815912
[31] Gill CO. Meat spoilage and evaluation of the potential storage life of fresh meat. J Food Prot. 1983;46(5):444-52. DOI: 10.4315/0362-028X-46.5.444
[32] Nychas GJE, Skandamis PN, Tassou CC, Koutsoumanis KP. Meat spoilage during distribution. Meat Sci. 2008;78(1-2):77-89. DOI: 10.1016/j.meatsci.2007.06.020
[33] Dainty RH, Mackey BM. The relationship between the phenotypic properties of bacteria from chill-stored meat and spoilage processes. J Appl Microbiol. 1992;73(s21):103s-114s. DOI: 10.1111/j.1365-2672.1992.tb03630.x
[34] Russell SM, Fletcher DL, Cox NA. Spoilage bacteria of fresh broiler chicken carcasses. Poult Sci. 1995 Dec;74(12):2041-7. DOI: 10.3382/ps.0742041
[35] Borch E, Kant-Muermans ML, Blixt Y. Bacterial spoilage of meat and cured meat products. Meat Sci. 1996;33(1):103-20. DOI: 10.1016/0168-1605(96)01135-X
[36] Erkmen O, Bozoglu TF. Spoilage of meat and meat products. In: Erkmen O, Bozoglu TF, editors. Food microbiology: principles into practice. Chichester: John Wiley & Sons; 2016. p. 279-95.
[37] Iulietto MF, Sechi P, Borgogni E, Cenci-Goga BT. Meat spoilage: a critical review of a neglected alteration due to ropy slime producing bacteria. Ital J Anim Sci. 2016;14:4011. DOI: 10.4081/ijas.2015.4011
[38] Mossel DAA. Physiological and metabolic attributes of microbial groups associated with foods. J Appl Bacteriol. 1971;34:95-118.
[39] Bolder NM. The microbiology of the slaughter und processing of poultry. In: Davies AR, Board R, editors. The microbiology of meat and poultry. London: Blackie Academic & Professional; 1998. p. 158-73.
[40] Rouger A, Tresse O, Zagorec M. Bacterial contaminants of poultry meat: sources, species, and dynamics. Microorganisms. 2017;5(3):50. DOI: 10.3390/microorganisms5030050
[41] Lues JFR, Theron MM, Venter P, Rasephei MHR. Microbial composition in bioaerosols of a highthroughput chicken-slaughtering facility. Poult Sci. 2007;86:142-9. DOI: 10.1093/ps/86.1.142
[42] Blickstad E, Molin G. The microbial flora of smoked pork loin and frankfurter sausage stored in different gas atmospheres at 4°C. J Appl Bact. 1983;54(1):45-56. DOI: 10.1111/j.1365-2672.1983.tb01299.x
[43] Mead GC. Microbiological quality of poultry meat: a review. Rev Bras Cienc Avic. 2004;6(3):135-42. DOI: 10.1590/S1516-635X2004000300001
[44] Nørrung B, Buncic S. Microbial safety of meat in the European Union. Meat Sci. 2008;78(1-2):14-24. DOI: 10.1016/j.meatsci.2007.07.032
[45] Gram L, Ravn L, Rasch M, Bruhn JB, Christensen AB, Givskov M. Food spoilage – interactions between food spoilage bacteria. Int J Food Microbiol. 2002;78(1-2):79-97. DOI: 10.1016/S0168-1605(02)00233-7
[46] McDonald K, Sun DW. Predictive food microbiology for the meat industry: a review. Int J Food Microbiol. 1999;52(1-2):1-27. DOI: 10.1016/S0168-1605(99)00126-9
[47] Raab V, Bruckner S, Beierle E, Kampmann Y, Petersen B, Kreyenschmidt J. Generic model for the prediction of remaining shelf life in support of cold chain management in pork and poultry supply chains. J Chain Netw Sci. 2008;8(1):59-73. DOI: 10.3920/JCNS2008.x089
[48] Bruckner S, Albrecht A, Petersen B, Kreyenschmidt J. Characterization and comparison of spoilage processes in fresh pork and poultry. J Food Qual. 2012;35(5):372-82. DOI: 10.1111/j.1745-4557.2012.00456.x
[49] Henchion M, McCarthy M, Resconi VC, Troy D. Meat consumption: trends and quality matters. Meat Sci. 2014;98(3):561-8. DOI: 10.1016/j.meatsci.2014.06.007
[50] Verbeke W, Pérez-Cueto FJA, de Barcellos MD, Krystallis A, Grunert KG. European citizen and consumer attitudes and preferences regarding beef and pork. Meat Sci. 2010;84(2):284-92. DOI: 10.1016/j.meatsci.2009.05.001
[51] Kreyenschmidt J, Ibald R. Modeling shelf life using microbial indicators. In: Nicoli MC, editor. Shelf life assessment of food. Boca Raton: CRC Press; 2012.
[52] Cameron ND. Genetic and phenotypic parameters for carcass traits, meat and eating quality traits in pigs. Livestock Production Science. 1990;26(2):119-35. DOI: 10.1016/0301-6226(90)90061-A
[53] Kuttappan VA, Brewer VB, Apple JK, Waldroup PW, Owens CM. Influence of growth rate on the occurrence of white striping in broiler breast fillets. Poult Sci. 2012;91(10):2677-85. DOI: 10.3382/ps.2012-02259
[54] Barbut S, Sosnicki AA, Lonergan SM, Knapp T, Ciobanu DC, Gatcliffe LJ, et al. Progress in reducing the pale, soft and exudative (PSE) problem in pork and poultry meat. Meat Sci. 2008;79(1):46-63. DOI: 10.1016/j.meatsci.2007.07.031
[55] Dransfield E, Sosnicki A. Relationship between muscle growth and poultry meat quality. Poult Sci. 1999;78(1):743-6. DOI: 10.1093/ps/78.5.743
[56] Le Bihan-Duval E, Debut M, Berri CM, Sellier N, Sante-Lhoutellier V, Jégo Y, Beaumont C. Chicken meat quality: genetic variability and relationship with growth and muscle characteristics. BMC Genet. 2008;9:53. DOI: 10.1186/1471-2156-9-53
[57] Monin G, Mejenes-Quijano A, Talmant A. Influence of breed and muscle metabolic type on muscle glycolytic potential and meat pH in pigs. Meat Sci. 1987;20:149-58.
[58] Schwörer D, Blum J, Rebsamen A. Parameters of meat quality and stress resistance of pigs. Livest Prod Sci. 1980;7:337-48.
[59] Ferguson DM, Warner RD. Have we underestimated the impact of pre-slaughter stress on meat quality in ruminants? Meat Sci. 2008;80(1):12-9. DOI: 10.1016/j.meatsci.2008.05.004
[60] Debut M, Berri C, Baeza E, Sellier N, Arnould C, Guemene D, et al. Variation of chicken technological meat quality in relation to genotype and preslaughter stress conditions. Poultry Sci. 2003;82(12):1829-38. DOI: 10.1093/ps/82.12.1829
[61] Rosenvold K, Andersen HJ. Factors of significance for pork quality – a review. Meat Sci. 2003;64(3):219-37. DOI: 10.1016/S0309-1740(02)00186-9
[62] Leach LM, Ellis M, Sutton DS, McKeith FK, Wilson ER. The growth performance, carcass characteristics, and meat quality of halothane carrier and negative pigs. J Anim Sci. 1996 May;74(5):934-43. DOI: 10.2527/1996.745934x
[63] Rosenvold K, Andersen HJ. The significance of pre-slaughter stress and diet on colour and colour stability of pork. Meat Sci. 2003;63:199-209. DOI: 10.1016/S0309-1740(02)00071-2
[64] Pettigrew JE, Esnaola MA. Swine nutrition and pork quality: a review. J Anim Sci. 2001;79:E316-E342.
[65] Andersen HJ, Oksbjerg N, Young JF, Therkildsen M. Feeding and meat quality - a future approach. Meat Sci. 2005;70(3):543-54. DOI: 10.1016/j.meatsci.2004.07.015
[66] Guardia S, Lessire M, Corniaux A, Métayer-Coustard S, Mercerand F, Tesseraud S, et al. Short-term nutritional strategies before slaughter are effective in modulating the final pH and color of broiler breast meat. Poultry Sci. 2014;93(7):1764-73. DOI: 10.3382/ps.2013-03768
[67] Wen C, Jiang XY, Ding LR, Wang T, Zhou YM. Effects of dietary methionine on growth performance, meat quality and oxidative status of breast muscle in fast- and slow-growing broilers. Poultry Sci. 2016;96(6):1707-14. DOI: 10.3382/ps/pew432
[68] Wallis IR. Dietary supplements of methionine increase breast meat yield and decrease abdominal fat in growing broiler chickens. Aust J Exp Agric. 1999;39(2):131-41. DOI: 10.1071/EA98130
[69] Berri C, Besnard J, Relandeau C. Increasing dietary lysine increases final pH and decreases drip loss of broiler breast meat. Poultry Sci. 2008;87(3):480-4. DOI: 10.3382/ps.2007-00226
[70] Fanatico AC, Owens CM, Emmert JL. Organic poultry production in the United States: Broilers. J Appl Poultry Res. 2009;18(2):355-66. DOI: 10.3382/japr.2008-00123
[71] Castellini C, Mugnai C, Dal Bosco A. Effect of organic production system on broiler carcass and meat quality. Meat Sci. 2002;60(3):219-25. DOI: 10.1016/S0309-1740(01)00124-3
[72] Fanatico AC, Pillai PB, Emmert JL, Owens CM. Meat quality of slow- and fast-growing chicken genotypes fed low-nutrient or standard diets and raised indoors or with outdoor access. Poultry Sci. 2007;86(10):2245-55. DOI: 10.1093/ps/86.10.2245
[73] Mikulski D, Celej J, Jankowski J, Majewska T, Mikulska M. Growth performance, carcass traits and meat quality of slower-growing and fast-growing chickens raised with and without outdoor access. Asian Australas J Anim Sci. 2011;24(10):1407-16. DOI: 10.5713/ajas.2011.11038
[74] De Jonge J, van Trijp HCM. Meeting heterogeneity in consumer demand for animal welfare: a reflection on existing knowledge and implications for the meat sector. J Agric Environ Ethics. 2013;26:629-61. DOI: 10.1007/s10806-012-9426-7
[75] Bessei W. Welfare of broilers: a review. Worlds Poult Sci J. 2006;62(3):455-66. DOI: 10.1017/S0043933906001085
[76] Klauke TN, Pineiro M, Schulze-Geisthovel S, Plattes S, Selhorst T, Petersen B. Coherence of animal health, welfare and carcass quality in pork production chains. Meat Sci. 2013;95(3):704-11. DOI: 10.1016/j.meatsci.2013.03.022
[77] Rocha LM, Velarde A, Dalmau A, Saucier L, Faucitano L. Can the monitoring of animal welfare parameters predict pork meat quality variation through the supply chain (from farm to slaughter)? J Anim Sci. 2016;94(1):359-76. DOI: 10.2527/jas.2015-9176
[78] Wilson PD, Brocklehurst TF, Arino S, Thuault D, Jakobsen M, Lange M, et al. Modelling microbial growth in structured foods: towards a unified approach. Int J Food Microbiol. 2002 Mar;73(2-3):275-89. DOI: 10.1016/s0168-1605(01)00660-2
[79] Robins MM, Wilson PDG. Food structure and microbial growth. Trends Food Sci Technol. 1994;5(9):289-93. DOI: 10.1016/0924-2244(94)90137-6
[80] Esener AA, Bol G, Kossen NWF, Roels JA. Effect of water activity on microbial growth. In: Moo-Young M, Robinson CW, Vezina C, editors. Scientific and engineering principles. Toronto: Pergamon; 1981. p. 339-44. DOI: 10.1016/B978-0-08-025383-1.50062-X
[81] Leistner L, Rödel W. The significance of water activity for micro-organisms in meats. In: Duckworth R, editor. Water relations of foods: proceedings of an international symposium held in Glasgow, September 1974. London: Academic Press; 1975. p. 309-23. DOI: 10.1016/B978-0-12-223150-6.50022-5
[82] Gibson AM, Bratchell N, Roberts TA. Predicting microbial growth: growth responses of salmonellae in a laboratory medium as affected by pH, sodium chloride and storage temperature. Int J Food Microbiol. 1988;6(2):155-78. DOI: 10.1016/0168-1605(88)90051-7
[83] Gill CO, Newton KG. The ecology of bacterial spoilage of fresh meat at chill temperatures. Meat Sci. 1978 Jul;2(3):207-17. DOI: 10.1016/0309-1740(78)90006-2
[84] Guàrdia MD, Estany J, Balasch S, Oliver MA, Gispert M, Diestre A. Risk assessment of DFD meat due to pre-slaughter conditions in pigs. Meat Sci. 2010;70(4):709-16. DOI: 10.1016/j.meatsci.2005.03.007
[85] Newton KG, Gill CO. The microbiology of DFD fresh meats: a review. Meat Sci. 1981 May;5(3):223-32. DOI: 10.1016/0309-1740(81)90005-X
[86] Barbut S, Zhang L, Marcone M. Effects of pale, normal, and dark chicken breast meat on microstructure, extractable proteins, and cooking of marinated fillets. Poultry Sci. 2005;84(1):797-802. DOI: 10.1093/ps/84.5.797
[87] Gajana CS, Nkukwana TT, Marume U, Muchenje V. Effects of transportation time, distance, stocking density, temperature and lairage time on incidences of pale soft exudative (PSE) and the physico-chemical characteristics of pork. Meat Sci. 2013;95(3):520-5. DOI: 10.1016/j.meatsci.2013.05.028
[88] Coates KJ, Beattie JC, Morgan IR, Widders PR. The contribution of carcass contamination and the boning process to microbial spoilage of aerobically stored pork. Food Microbiol. 1995;12:49-54. DOI: 10.1016/S0740-0020(95)80078-6
[89] Zhou GH, Xu XL, Liu Y. Preservation technologies for fresh meat – a review. Meat Sci. 2010;86(1):119-28. DOI: 10.1016/j.meatsci.2010.04.033
[90] Dempster JF. Radiation preservation of meat and meat products: a review. Meat Sci. 1985;12(2):61-89. DOI: 10.1016/0309-1740(85)90017-8
[91] Andrews LS, Ahmedna M, Grodner RM, Liuzzo JA, Murano PS, Murano EA, et al. Food preservation using ionizing radiation. Rev Environ Contam Toxicol. 1998;154:1-53. DOI: 10.1007/978-1-4612-2208-8_1
[92] Labadie J. Consequences of packaging on bacterial growth - meat is an ecological niche. Meat Sci. 1999 Jul;52(3):299-305. DOI: 10.1016/s0309-1740(99)00006-6
[93] McMillin KW. Advancements in meat packaging. Meat Sci. 2017;132:153-62. DOI: 10.1016/j.meatsci.2017.04.015
[94] Herbert U, Albrecht A, Kreyenschmidt J. Definition of predictor variables for MAP poultry filets stored under different temperature conditions. Poultry Sci. 2015;94(1):424-32. DOI: 10.3382/ps/peu002
[95] Herbert U. Assessment of different packaging atmospheres for the poultry meat industry based on an overall quality index [dissertation]. Bonn: University of Bonn; 2014. 126 p. Available from: https://d-nb.info/1077269102/34
[96] McMillin KW. Where is MAP going? A review and future potential of modified atmosphere packaging for meat. Meat Sci. 2008;80(1):43-65. DOI: 10.1016/j.meatsci.2008.05.028
[97] Kerry JP, O’Grady MN, Hogan SA. Past, current and potential utilisation of active and intelligent packaging systems for meat and muscle-based products: a review. Meat Sci. 2006;74(1):113-30. DOI: 10.1016/j.meatsci.2006.04.024
[98] Leinonen I, Kyriazakis I. How can we improve the environmental sustainability of poultry production? The Proc Nutr Soc. 2016;75(3):265-73. DOI: 10.1017/S0029665116000094
[99] Castellini C, Boggia A, Cortina C, Dal Bosco A, Paolotti L, Novelli E, Mugnai C. A multicriteria approach for measuring the sustainability of different poultry production systems. J Clean Prod. 2012;37:192-201. DOI: 10.1016/j.jclepro.2012.07.006
[100] Magdelaine P, Spiess MP, Valceschini E. Poultry meat consumption trends in Europe. World’s Poult Sci J. 2008;64(1):53-64. DOI: 10.1017/S0043933907001717
[101] Borell Ev, Sørensen JT. Organic livestock production in Europe: aims, rules and trends with special emphasis on animal health and welfare. Livest Prod Sci. 2004;90(1):3-9. DOI: 10.1016/j.livprodsci.2004.07.003
[102] Trienekens J, Petersen B, Wognum N, Brinkmann D. European pork chains. Wageningen, the Netherlands: Wageningen Academic Publishers; 2009.